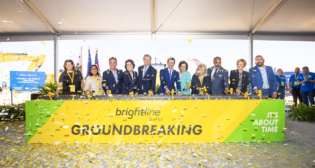
Timeout for Tech: The Invisible Materials Energy Component
Written by Gary T. Fry, Vice President, Fry Technical Services, Inc.; Railway Age Contributing Editor
Figure 1. A stretched rubber band contains energy that we call “strain energy.” One component of strain energy is the “strain” in the band, which manifests as the band’s visible stretch. The other component of strain energy is “stress” which is invisible. (All illustrations courtesy of Gary T. Fry)
RAILWAY AGE, OCTOBER 2023 ISSUE: What exactly is stress? It’s a tough—and often-asked—question.
Welcome to “Timeout for Tech with Gary T. Fry, Ph.D., P.E.” Each month, we examine a technology topic about which professionals in the railway industry have asked to learn more. This month our subject is stress in engineering materials.
“What is stress?” This is unarguably the most common question that I have been asked over the years. And it’s a tough one!
Various concepts of stress in structural materials have been with us for more than five centuries, beginning with Leonardo da Vinci in the 1490s. By the early 1820s, Augustin-Louis Cauchy had mathematically formalized a definition of stress that we now take for granted. In the 300 years between da Vinci and Cauchy, many famous scholars worked on what today would be considered problems in stress analysis, including Galileo, Hooke, Newton, members of the Bernoulli family, Euler and many others.
Today it is Cauchy’s theory of stress that is most commonly implemented to perform a stress analysis of engineering materials under load, such as steel railway rails, wheels and bridges. This makes our modern understanding of stress in materials slightly more than 200 years old—roughly as old as the first railways.
So, what is stress? Consider Figure 1 (above), which is a photograph of a stretched rubber band. Everyone understands that this system contains energy just waiting to be released at an opportune moment. In mechanics of materials, we call this energy strain energy, and stress is one of its two constituent factors—the other being strain.
Stress quantifies the intensity of internal forces that are distributed throughout a material when it is loaded. Stress is the component of strain energy that will cause the material to break, and that is why it is such an important concept. In fact, it was the desire to understand failures of structural components, such as beams, that originally led da Vinci, Galileo and others to our earliest concepts of stress.
In Figure 1, stress is the force in the rubber band per unit of cross-sectional area of the band and has units of pressure, for example pounds per square inch (PSI). Stress is invisible, and though it is never directly measurable, it can be calculated using appropriate mathematical expressions. In Figure 1, strain is the stretch in the band per unit length of the band and is effectively dimensionless with units of inches per inch (in./in.), for example. Strain is always manifest in the visible deformation of a material and can be measured.
Cauchy’s definition of stress allows us to quantify the strength of a material by testing the material to failure and performing a stress analysis to determine the level of stress that caused the failure. Subsequently stress analysis allows us to ensure we are applying load to a material safely. We do this by limiting the maximum stresses in a material to levels reliably below its strength. That is why experts sometimes remark that a failed component was “overstressed.”
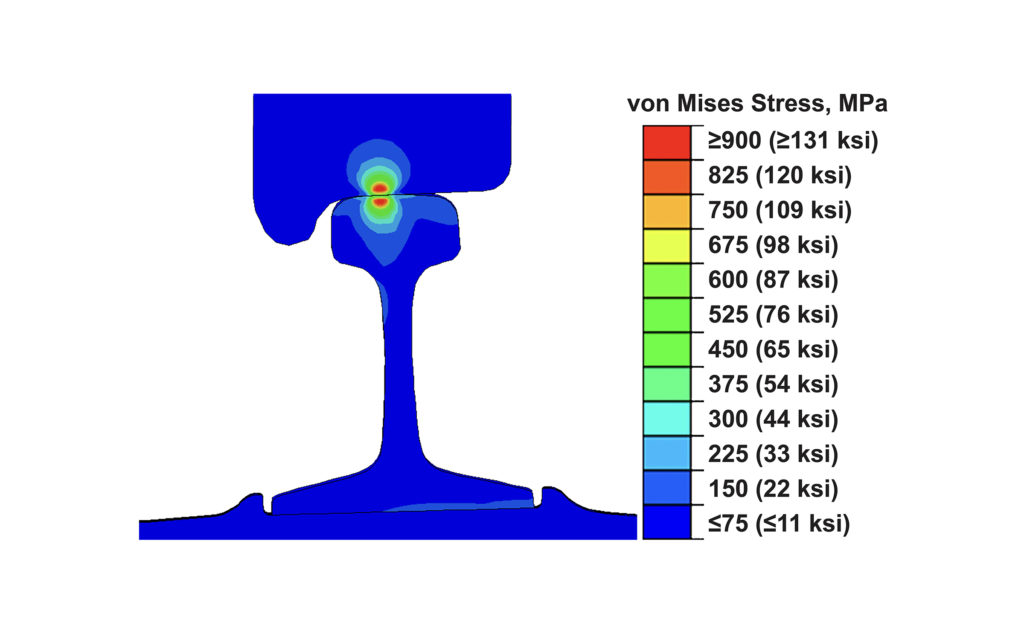
Figure 2. Contour plot of stresses from an analysis of wheel/rail contact.
In summary, stress is a mathematical concept that solves a set of partial differential equations. Stress in materials is invisible and not directly measurable. Modern stress analysis techniques allow us to accurately estimate three-dimensional stresses in many types of components under many types of complex loadings. For example, Figure 2 shows a contour plot of stresses calculated from an analysis of wheel/rail contact. Figure 3 shows a contour plot of stresses calculated from an analysis of a steel railway bridge loaded by a train.
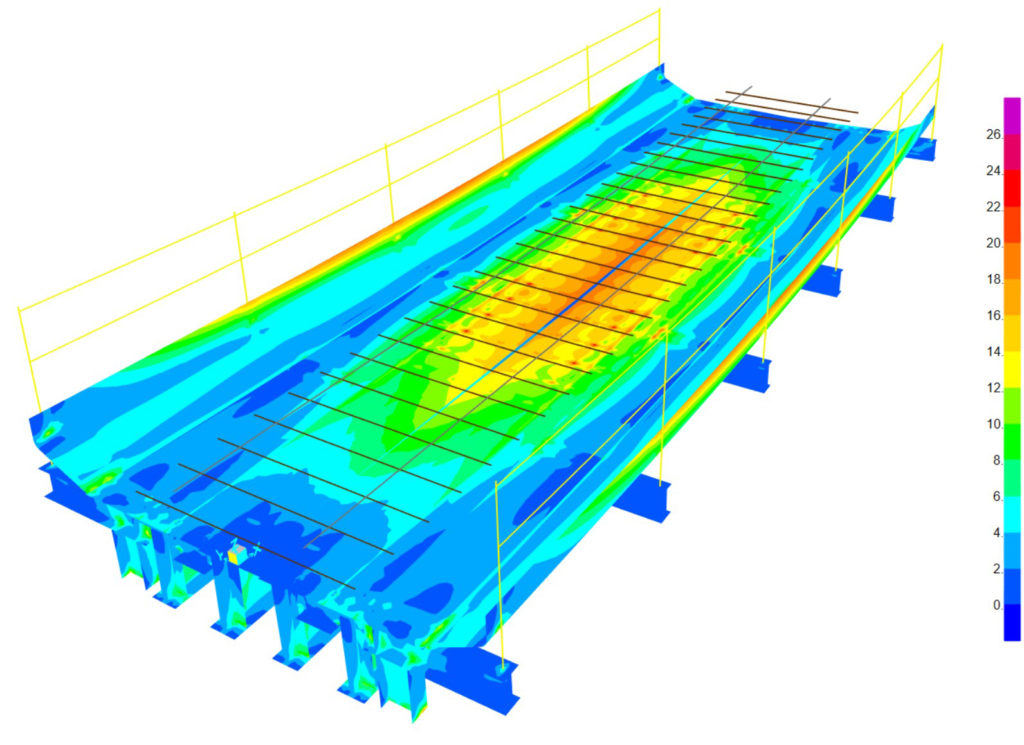
Figure 3. Contour plot of stresses from an analysis of a railway bridge.
Five-hundred years ago, an idea was first conceived that a loaded material was subjected to stress that might be quantified based on the geometry of the material and the nature of the loads applied to it. Now, stress analysis is a routine and critical part of ensuring the safety of the engineered systems we depend on every day.
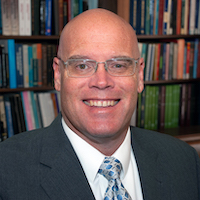
Dr. Fry is Vice President of Fry Technical Services, Inc. (https://www.frytechservices.com/). He has 30 years of experience in research and consulting on the fatigue and fracture behavior of structural metals and weldments. His research results have been incorporated into international codes of practice used in the design of structural components and systems, including structural welds, railway and highway bridges, and high-rise commercial buildings in seismic risk zones. He has extensive experience performing in-situ testing of railway bridges under live loading of trains, including high-speed passenger trains and heavy-axle-load freight trains. His research, publication, and consulting have advanced the state of the art in structural health monitoring and structural impairment detection.